Positrons: How antimatter helps doctors fight cancer
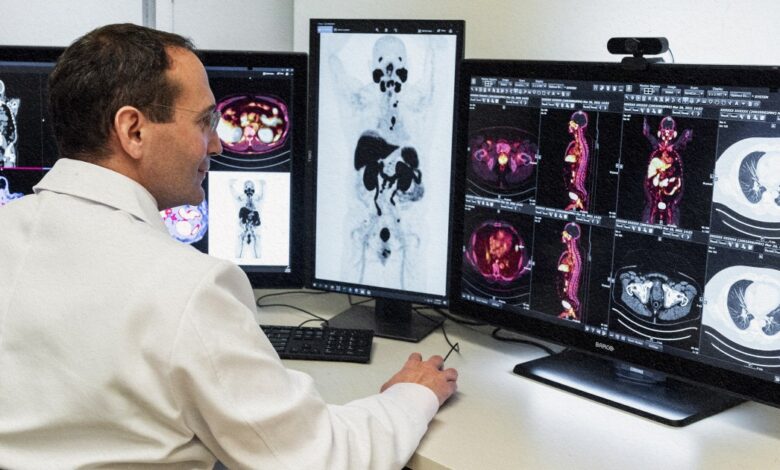
“You have cancer” is one of the scariest phrases you might ever hear. Luckily, in this modern age, we have a dizzying array of fantastic diagnostic, imaging, and treatment tools, many of which have arisen from our mastery of atomic and subatomic physics. However, there is one that seems almost too futuristic to be real — a technique that relies on antimatter inside the human body. This technique is called a positron emission tomography (PET) scan.
Antimatter
Positrons are the antimatter equivalent of negatively charged electrons. They have the same mass as electrons but an opposite (positive) electrical charge.
Antimatter sounds like science fiction. It is said to power the warp engines of the fictional Starship Enterprise. In Dan Brown’s book Angels and Demons, scientists working at the CERN laboratory in Europe isolated a quarter gram of antimatter with which they intended to destroy the Vatican. But antimatter is indeed quite real. It was predicted in 1928 and discovered a short four years later.
Antimatter is potentially dangerous. Combine equal amounts of matter and antimatter, and a tremendous amount of energy is released as they annihilate each other. In fact, combining a gram of matter and antimatter would release the energy of the nuclear detonations at Hiroshima and Nagasaki combined.
So, how can such a dangerous substance be a useful diagnostic tool for a deadly disease like cancer? And how can it exist inside the body, let alone be used safely? Just how does a PET scan work?
Positron emission
Antimatter is used indirectly. There are different kinds of radioactivity, and one rare form is called positron emission, which occurs when the proton inside an atomic nucleus decays, transforming itself into a neutron, a positron, and a neutrino. This type of decay is almost never seen in nature but occurs in a handful of manmade isotopes. (Isotopes are forms of familiar chemical elements, with an unusual number of neutrons in the nucleus, often making them unstable.) These isotopes are made by shooting a particle beam at common elements, which can cause them to transform into the unstable isotopes.
These positron-emitting isotopes live for a very short time, typically a few tens of minutes. One of the most long-lived is fluorine-18, which has a half-life of about 110 minutes. (A half-life is the time required for half of the radioactive isotopes to decay.) The exact isotope used in a medical setting depends on what the doctors are looking for.
For example, a common substance used in PET scanning is fluorodeoxyglucose (18F-FDG), with the fluorine atom being a fluorine-18 isotope. 18F-FDG is a glucose analog that is absorbed by tissues in the body, but it cannot be metabolized by cells, instead just building up inside of them. Cancer cells are especially prone to absorbing 18F-FDG, which allows doctors to look for places in the body where there is a high concentration of it.
To determine where the 18F-FDG is concentrated, doctors look for the emission of a positron when 18F decays. Being antimatter, however, the positron doesn’t make it very far. When the positron encounters an electron, they annihilate each other, and their masses are transformed into two gamma-rays with very specific energies. The rays travel in opposite directions, and a detector that surrounds the entire body detects them. Computers use the trajectories to locate from where in the body the positron was emitted.
Over the course of 10 or 20 minutes, perhaps a million positrons are emitted. By drawing line after line, computers can determine where the lines cross — and these are the candidate cancer clusters.
PET scan problems
PET is a powerful tool in the bag of tricks used by oncologists to monitor whether cancers are metastasizing or in remission, but the technology has some drawbacks. Because of the specialized tools needed to create the radioactive isotopes, not all hospitals can offer PET scanning. The very short half-life of many positron-emitting isotopes means that the isotopes must be created near where they are used, which sometimes means that the hospital has a particle accelerator on site. And because a particle accelerator can damage tracer molecules (like fluorodeoxyglucose), they cannot be synthesized until after the isotopes are made. Also, PET scans are not as precise at locating cancers as MRI or CT.