Plasma and the strange states of matter made of broken atoms
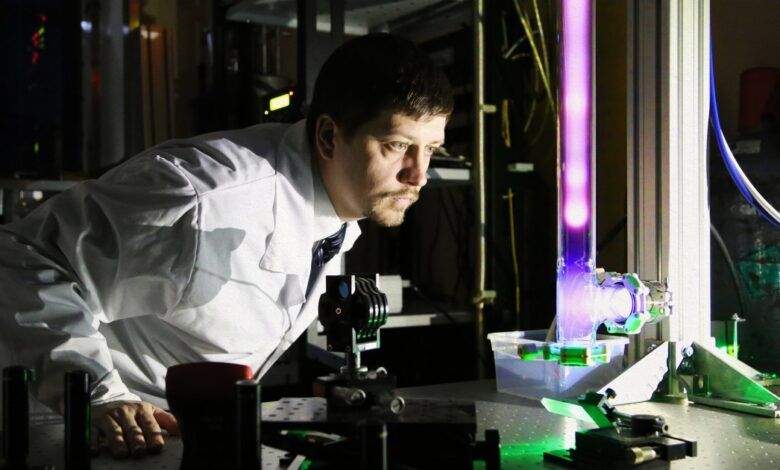
Nearly all matter with which we interact on a daily basis is in one of three simple states. Our largely liquid bodies stand on solid ground and breathe air made of gas. But there are many more than just these three, some of which are very strange and created by breaking apart atoms.
The usual states of matter
A solid is typically the least energetic form of any particular matter. Cool any type of matter toward absolute zero, and somewhere along the way, it will almost always condense into a solid form. Add heat and the solid will melt into a liquid. Its atoms are freed from their locked positions to roam about, but they aren’t so free as to fly away. Keep adding heat (and/or lower the pressure), and the liquid boils into a gas. The particles that constitute gaseous matter have enough energy to leave their fellow particles behind and explore the limits of their confinement.
In these states, each atom remains intact: Electrons surround nuclei made of protons and neutrons. But adding energy or applying pressure can break down atoms to reveal new states of matter.
Plasma
The simplest is plasma, in which electrons are stripped away from their nuclei. Think of glowing neon signs. Inside those glowing tubes is an ionized gas of positively charged atoms (ions) and free electrons, bathed in a flux of photons emitted by electrons bouncing between different energy levels.
A gas can be ionized into plasma through heating. At high temperature, collisions between speeding atoms are violent enough to kick electrons away from nuclei. Plasma also can be created by putting gas into a strong electrical field and shooting electrons at it. The freed electrons are accelerated by the electric field, which turns them into additional bullets, impacting more atoms and freeing yet more electrons — similar to a nuclear chain reaction.
Plasma is not particularly common on the surface of Earth, but it is in space. The Sun is predominantly made of plasma, as are some layers of Earth’s upper atmosphere. The ionosphere is named after its ionized gas. Within it, a hot region called the thermosphere produces visible auroras. Plasma in each of these systems is often known for the brilliant light of its photons.
Quark-gluon plasma
Freeing an electron from an atom is relatively simple compared to disassembling its nucleus. But this can be done, too, and the result is a quark-gluon plasma.
High-energy particle experiments smash neutrons and protons together, and they fragment into smaller particles called quarks. When quarks are separated from each other, tiny force-carrying particles called gluons fly between them. Like the plasma described above, quarks are analogous to the positively charged particles, while glowing gluons are analogous to photons.
It is difficult to describe this strange state of matter because it is so far removed from human experience. Tiny pockets of it are created at giant particle accelerators. Quark-gluon plasma has a temperature so high — trillions of degrees — that physicists struggle to figure out how to even measure it, and the quark-gluon plasma disappears in less than a billionth of a trillionth of a second. In nature, quark-gluon plasmas likely only existed in the early moments after the Big Bang.
Degenerate matter
There’s other weird stuff out there. White dwarfs and neutron stars are made of degenerate matter. A typical atom has a miniscule nucleus containing nearly all its mass, surrounded by a great deal of nearly empty space populated by a thin fog of featherweight electrons. Degenerate matter collapses this empty space and packs what remains with particles.
While plasmas liberate subatomic pieces of matter from their bonds to one another, degenerate matter confines them in a terribly crowded prison. It forms as regular matter is crushed under unimaginable pressure, squeezed until it collapses. The gravitational force present in an imploding star is so great that it overwhelms all resistance except the final quantum mechanical barriers holding subatomic particles apart. (At this point, the matter is only one step away from becoming a black hole, where gravity overwhelms even quantum mechanics.)
A white dwarf is a star made of electron degenerate matter. A star of similar mass to our Sun, it has run through its life cycle stages — yellow dwarf, red giant, and so forth — and exhausted the last of its fuel for fusion energy. No longer able to sustain itself, the star subsequently implodes. The ingenious astrophysicist Subrahmanyan Chandrasekhar calculated that the resistance of electrons to being packed together, governed by quantum mechanics, could halt the collapse before it reached a black hole state. Chandrasekhar concluded that white dwarf material was so dense that one cup might weigh several hundred tons.
Neutron stars are under even more extreme pressure. They contain more mass than white dwarfs, and their stronger gravity overwhelms the ability of electron degenerate matter to hold against its pressure. The electrons and nuclei are crushed into a single solid mass. Negatively charged electrons and positively charged protons are pushed together, creating additional neutrons. Total gravitational collapse is staved off only by the packed neutrons quantum mechanically repelling one another. While a baseball weighs a few ounces, a baseball-sized chunk of a neutron star weighs roughly 1017 grams — about one hundred trillion pounds.
There may be stranger objects out there still, including hypothetical exotic stars. A quark star would be a neutron star in which gravity has squished neutrons into their component quarks. If the repulsion between quarks is enough to stave off gravity, this object might hold steady. At still greater pressures, theory suggests that some quarks would become strange quarks, forming strange matter and creating a strange star. But these speculative degenerate matter stars have not been observed convincingly by astrophysicists.