How Earth’s eerie “sodium glow” helps us image stars

Adaptive optics (AO) are required for giant telescopes on Earth’s surface. (For a brief intro on the topic, see our previous article.) Their enormous curved mirrors collect a great amount of light which is blurred by transit through the atmosphere. World-class 300″ to 400” telescopes such as Keck, Subaru, Gran Telescopio Canarias, the Very Large Telescope, and the upcoming Great Magellan Telescope all use AO. These systems analyze the telescope’s image in real-time and then actively warp their mirrors to counteract its blurring.
The computer that controls these systems has to find a reference point that is not distorted, against which to compare the blurred image. But how? The answer lies in the twinkle and shimmer of the stars that we can see with our naked eyes because behind every twinkling, slightly blurry speck is a nearly perfect stationary light source.
Earth’s sodium layer
Astronomers can create and measure a shimmering artificial “guide star” with a precisely known shape and position. They accomplish this by taking advantage of the naturally existing sodium in our upper atmosphere. This thin layer is a fascinating thing by itself. The sodium is likely formed by meteor “ablation” — in other words, literally blasted off the surface of space rocks as they pass through the Earth’s atmosphere. There is some debate on the details driving it. Regardless, it’s observably there. The ghostly orange glow characteristic of the sodium layer can be seen in beautiful images taken from the international space station.

The sodium atoms emit — and thus also absorb — light at a wavelength near 589 nm (nanometers), which we perceive as a yellow-orange color. To create an artificial star, the telescope shines a laser at this same wavelength up into the night sky. The concentrated beam passes through the nearly transparent atmosphere mostly undisturbed until reaching the sodium layer, centered at about 90 km (56 mi) in altitude and roughly 20 km (12 mi) thick. It contains plenty of sodium atoms — a few billion per cubic meter — though even at this height, they make up only a tiny fraction of the thin air.
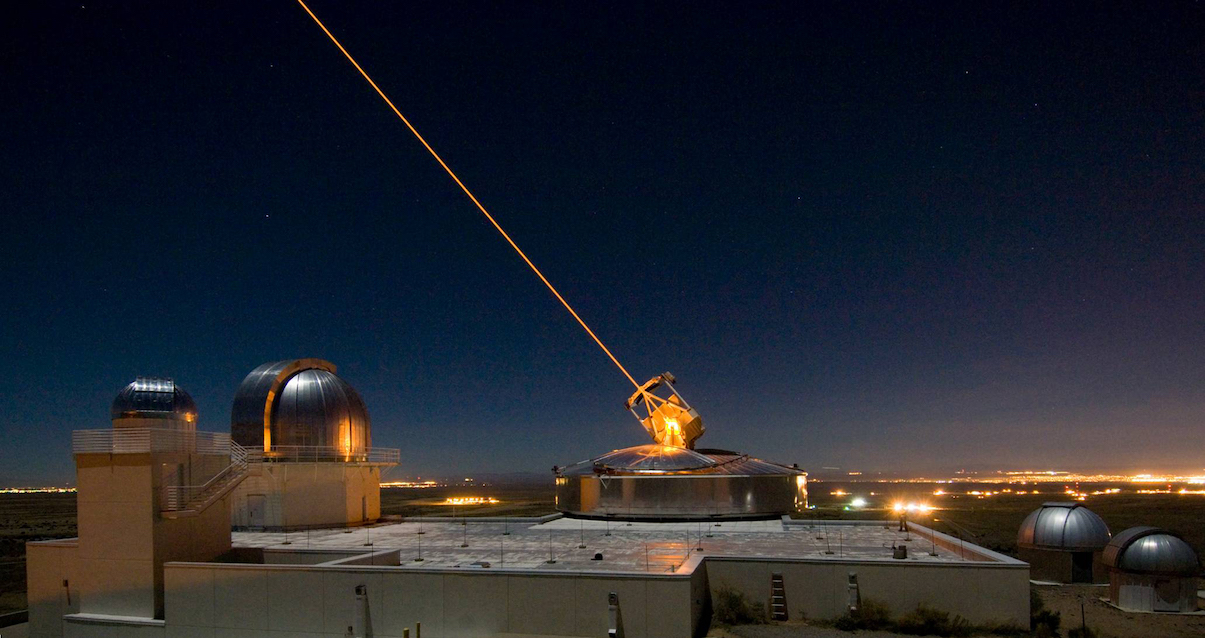
Within the layer, sodium atoms will periodically absorb the laser photons along the beam and then re-emit them in all directions like a star. This creates a cylinder of glowing light in the upper atmosphere. From the ground, looking straight up at the bottom of the long, but very thin cylinder, it looks just like a tiny circular star. (Because a long cylinder in the atmosphere looks like a line when viewed from the side, the solution is to mount the laser at the center of the scope.)
Adaptive optics in action
Whereas the light from a distant star comes down to the ground in parallel lines, the rays from the artificial guide star spread in a slightly conical way, so the guide star image is cleaned up by adjusting the telescope in a manner that counters the conical elongation. The resulting image is nearly static — unchanging in time — so that further adjustments are small. Once this basic adjustment is made, the AO system is ready to go live and counter dynamic — moving with time — atmospheric turbulence.
The sodium layer is high enough that the light emitted from the guide star must pass through nearly all of the atoms and molecules in the atmosphere. Pockets, gradients, and winds steer it into aberration. The blurred artificial starlight collected by the primary mirror is reflected off of a secondary mirror that is actively warped and bent by the AO system.
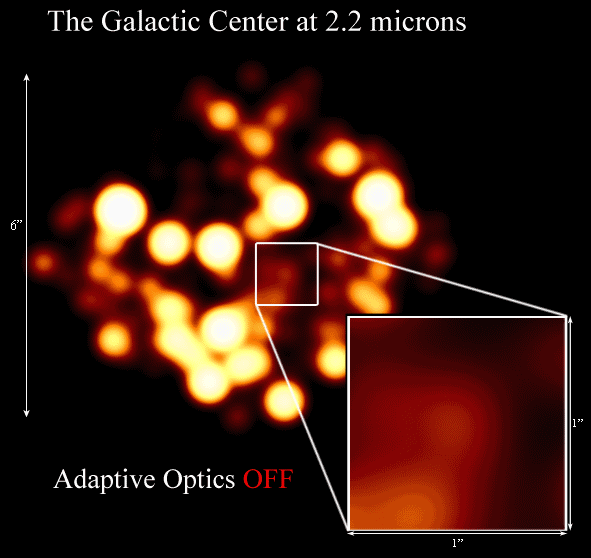
A small part of the light from the secondary mirror is split off, and its distortion is analyzed by a computer in real-time. The computer compares the measured guide star image to the ideal shape of the guide star and analyzes the apparent distortion according to modal or zonal theory (also explained in our previous AO story) at a rate of more than 1,000 times per second (or 1 kHz, expressed in units of frequency). The computer makes minute warping adjustments, at the same ~1 kHz rate, to keep the guide star shape perfectly correct. This de-blurs the telescope’s image of the sky near the guide star.
Correcting for distortion with the atmospheric sodium guide star, ground-based telescopes can achieve nearly unlimited resolution. By beating the limitations of the atmosphere, they are now constrained only by mirror size, with the attendant practical problems of funding, constructing, and caring for incredibly large mirrors that are impossibly smooth. In this way — for wavelengths of light that efficiently reach Earth’s surface, and are not confused with terrestrial sources — ground scopes with adaptive optics may eliminate the need for space telescopes.